The ability to illuminate select neurons within a population of optogenetic-expressing neurons is called cellular-resolution optogenetics (Shemesh et al. 2017). To use this technique, researchers require more sophisticated spatial specificity to selectively stimulate individual neurons within an optogenetic-expressing population.
The development of spatially targeted light technologies has enabled the control of where light illuminates the sample, such as an individual cell, making cellular-resolution optogenetics possible (Ronzitti et al. 2017).
What systems are available for cellular-resolution optogenetics?
Galvo-Scanning
Galvo-scanning uses a serial scanning method where a focused beam of light is directed to a specific region of the sample using a pair of galvanometer mirrors.
This “galvo-based scanning” technique typically uses a high-powered laser beam (either tunable or fixed-wavelength) for optogenetic stimulation. The illumination is restricted to a single spot at a time, and the intensity of the spot follows a Gaussian distribution. Patterns more complex than a single spot are traced out on the sample by illuminating different points sequentially, commonly by raster or spiral scanning, which are each useful for different experimental conditions.
As patterns are generated by serial scanning, the temporal precision of this system is primarily restricted by the positioning actuators of the galvanometer mirrors. Conventional galvo-based scanning systems take ~100 μs to redirect the beam to a new ROI. Consequently, pattern generation by scanning through the full field of view can take a significant amount of time. These limitations of temporal precision in this system present an issue for stimulating and recording fast physiological events, such as the generation of action potentials, which fire on the order of 100s of Hz.
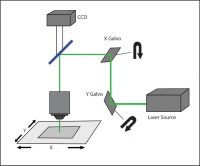
A galvo-scanning setup.
A key limitation of galvo-based scanning systems is that only a single, small spot can be illuminated at a given time. This inability to illuminate multiple regions of interest simultaneously fundamentally restricts the applications of the system, as it cannot address questions about how multiple neurons behave simultaneously.
The advantage of galvo-scanning is the minimal loss of light intensity along the optical pathway. Therefore, the focused beam offers good illumination intensity onto the sample, allowing the same system to be used for both light delivery and imaging. Furthermore, the illumination is fairly uniform and is not susceptible to background interference from other light sources.
Cellular-resolution optogenetics using a galvo-based scanning system is generally user-friendly and easy to integrate with existing systems and can be used for both in vitro and in vivo head-fixed experiments. While the galvanometer mirrors themselves are not particularly expensive, the requirement for high-powered lasers, which are a significant cost, and the software for controlling the galvos can lead to an expensive system.
Holographic Projection
Parallel excitation, as in phase and amplitude, spatial light modulation techniques may be used for cellular-resolution optogenetics. Parallel excitation methods are more adaptable to a variety of experimental needs, as they allow simultaneous illumination of multiple diffraction-limited spots on the sample.
Phase modulation commonly takes advantage of computer-generated holograms (CGHs) to stimulate multiple points at once. The desired illumination pattern is designed on a computer, after which the projection for patterned illumination is generated by a numerical algorithm that calculates the appropriate phase hologram. This hologram is then projected onto a spatial light modulator (SLM). A reference beam is reflected onto the SLM and through a set of imaging optics, to deliver a reconstructed illumination pattern onto the sample.
Holographic systems have the advantage of being able to stimulate any number and shape of three-dimensional patterns simultaneously onto the sample. Another advantage compared to galvo-based scanning is the holographic system offers far greater temporal resolution than serial scanning methods.
Holographic systems require up to several minutes to calculate and generate new patterns. This means for applications requiring quick or real-time pattern generation the holographic method would not be the most suitable. As the computation of new holographic patterns may take minutes to produce, this method is not suitable for applications requiring real-time pattern generation, uploading, and activation.
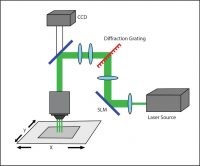
A holographic setup.
While this system theoretically achieves good illumination intensity, in practice, there are some efficiency concerns. Illumination spots are non-uniform, characterized by a bright focus with continuously diminishing intensity towards edges (Lutz et al. 2009). This results in poor contrast between illumination points and background and, hence, poorly defined edges of illumination.
The holographic system is the most costly and most difficult to integrate with existing systems. Holographic systems require a fair amount of expertise to use properly and can be very expensive, due in large part to the high cost of the parts (lasers and SLM, as well as computer hardware and software). There are also concerns with the lack of reliable precision and repeatability of the patterned illumination for the system, in particular with unintended speckling patterns. Holographic systems, therefore, require a significant level of expertise to create a working system for experimental use.
DMD Illuminator
A third system commonly used in optogenetic experiments employs a digital micromirror device (DMD), such as Mightex’s Polygon DMD Illuminator. A DMD is an array of many microscopic mirrors which can each be independently controlled.
When illuminated, each micromirror corresponds to a pixel in the illumination pattern that is defined by the user, and it can be individually and independently controlled to reflect light onto the sample. Mirrors are rotated approximately 12 degrees to either an on- or off-state (Knapcsyk & Krishnan 2005).
As with holographic pattern projection, DMD systems can stimulate multiple discrete ROIs simultaneously, allowing for parallel excitation of multiple cells while maintaining high contrast against the background. Because the micromirror array acts to reflect single pixels of light onto the sample, the illumination spot size on the sample is limited only by the objective lens and the number of micromirrors on the DMD chip.
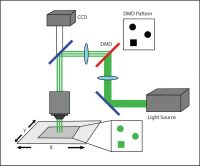
A DMD setup.
An advantage of pixelation with high contrast is that the illumination will be well contained within the pattern area. The sharp illumination edge helps ensure that light is only directed to the area of interest and that there is no spillover into unwanted regions. This can help provide more control to the excitation experiment.
A key advantage of the system is temporal precision (Wilt et al. 2009). Real-time pattern generation can also be achieved, and this is especially useful in closed-loop experiments where a moving target is being tracked and a precise illumination pattern must be generated and delivered to the target.
Unlike the galvo-scanner or the holographic projector, a DMD illuminator can be used with virtually any light source (e.g. lasers, LEDs, Xenon, metal halide, etc.) to illuminate the sample, making it a versatile system.
DMD-based systems have a high degree of illumination uniformity across the sample, meaning not only is uniformity maintained over a given illumination area, but also that intensity is consistent over discrete illumination areas, regardless of position in the field of view. Moreover, there is very little background noise (contrast ratio of 1000:1) to cause interference in the illumination, and no unwanted illumination patterns are generated, as is the case with galvo-scanning systems.
Typically DMD systems are most useful for studying the effect of activation of cell types in specific patterns (Blumhagen et al. 2011; Munch et al. 2009). DMDs have been widely used for in vitro optogenetics, as well as in vivo optogenetic experiments using organisms such as C. elegans (Leifer et al. 2011), zebrafish (Zhu et al. 2012), and mice, wherein optical access to the behaving animal is possible.
Of the three systems discussed in this paper, the DMD system offers the greatest ease of use at the lowest cost. It is easily integrated with existing commercial microscopes and is highly adaptable.
System Comparison Table
——————————
Related Products for Optogenetics