Published on 2024/02/29 Research powered by Mightex’s Polygon1000
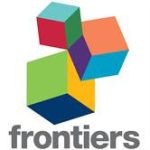
Tang, Y., Wu, Q., Gao, M., Ryu, E., Pei, Z., Kissinger, S. T., … & Chubykin, A. A., Restoration of visual function and cortical connectivity after ischemic injury through NeuroD1-mediated gene therapy. Frontiers in Cell and Developmental Biology, 9, 720078, (2021).
Is it possible to undo cell death in the brain? And can neural functions that are lost as a result of brain damage be regained? Tang et al (2021) explored this topic in their paper Restoration of Visual Function and Cortical Connectivity After Ischemic Injury Through NeuroD1-Mediated Gene Therapy.
In theory, a powerful approach to healing the brain following traumatic brain injury would be to induce neurogenesis at the site of the injury. If new neurons could appear in the injured region and then be reintegrated into the local network, it may allow for the regaining of lost function.
This, however, is not how the brain deals with brain injury in adult humans. Two barriers exist to the brain regenerating after localized damage. The first barrier is that neurogenesis only occurs in very specific regions of the brain in adult humans. The second problem is that, following traumatic brain injury, a scar composed of glial cells is formed at the site of the damage. Were the brain able to induce neurogenesis at the site of the injury, this scar would inhibit the new cells from taking the place of the old cells.
In this paper, Tang et al (2021) attempt to solve both problems in an elegant way – by converting the glial cells that compose the scar into neurons. This is done by introducing two adenoviruses into the injured region: GFAP::Cre, a construct that induces astrocytes to express Cre, and FLEX-NeuroD1-mCherry, a construct made to only target cells expressing Cre, simultaneously dyeing them with mCherry and initiating their conversion into neurons. When combined, these viruses ensure that any exposed astrocyte will be dyed with mCherry so it can be identified via fluorescent imaging, and converted into a neuron. The goal of this technique is to convert non-functional astrocytes into well integrated neurons. The researchers theorized that, if the neurons integrate properly, the region might recover from its trauma induced loss of function.
To investigate this technique, Tang et al. (2021) split 2.5 month old mice into 3 groups: a control group with a sham surgery, a second group with ischemia induced via endothelin (ET-1) expression in the primary visual cortex, and an experimental group with ischemia induced in the primary visual cortex and then, 2 weeks later, conversion of astrocytes into neurons in the traumatized region.
In their initial experiments, Tang et al (2021) explored the efficacy of the conversion using fluorescent labeling techniques. The researchers sacrificed a subset of each group at different time points along a 3 week period following exposure to NeuroD1. The researchers stained the tissue for GFAP, an astrocyte-specific marker, and NeuN, a neuron-specific marker. They found that, following NeuroD1 exposure, astrocytes initially only expressed GFAP but slowly began to express NeuN over the course of 3 weeks. 3 weeks following NeuroD1 injection, most cells that had previously expressed only GFAP now only expressed NeuN, indicating that the astrocytes had been converted to neurons (Figure 1).
Figure 1: Comparison of cell specific markers in astrocytes. White arrows represent cells co-expressing mCherry and GFAP. Yellow arrows represent cells co-expressing mCherry and Neun.
In a second experiment they investigated the connective properties of these reprogrammed neurons using a technique called channelrhodopsin assisted circuit mapping (CRACM). In this technique, channelrhodpisin (ChR2) is expressed in neurons in a sparse manner. After brain slicing, cells are randomly stimulated using a patterned illuminator. During the stimulation period, you can gain insight into the connectivity of cells of interest by patch clamping them.
In this CRACM experiment, Tang et al (2021) expressed both mCherry and NeuroD1 in astrocytes and ChR2 in pyramidal neurons in the visual cortex and then collected brain slices 3 weeks post-exposure. Then, a Polygon patterned light illuminator (Mightex) was used to illuminate individual pixels in the visual cortex in a random order. This illumination stimulated cells in the tissue and induced EPSCs across the layers of the visual cortex. The researchers then collected patch clamp readings from different cells of interest in the tissue and were able to create activity maps from different cell types across the groups. The researchers found that optogenetic stimulation of ChR2-positive cells induced high levels of activity in mCherry positive cells (Figure 2). Indeed, the activity from the reprogrammed cells was even higher than from cells in the healthy control; this is likely due to the newly formed neurons not having had their synaptic connections pruned. These findings indicate that the reprogrammed cells were being integrated into the visual cortex.
Figure 2: Comparison of CRACM maps from readings from patch clamped cells across groups.
Top Left: Averaged CRACM heat map for mCherry positive cells from the experimental group. Top Right: Averaged CRACM heat map for mCherry negative cells from the experimental group.
Bottom Right: Averaged CRACM heat map for cells from untreated ischemia control group.
Bottom Left: Averaged CRACM heat map for cells from healthy control.
In a final experiment, the researchers attempted to show, using calcium imaging, that the reprogrammed cells had not only integrated themselves in the visual circuit, but that they had become functional. To do this, the researchers reprogrammed the cells as before but this time expressed GCaMP in the reprogrammed cells. Next, they implanted GRIN lenses above the visual cortex and, after waiting for recovery, exposed the mice to a variety of visual stimuli. They were able to find that, similar to many pyramidal cells in the primary visual cortex, the cells had a stimulus specific firing. That is, these cells would only fire if the animal was shown a specific type of visual stimulus (Figure 3). The finding that the reprogrammed cells fired in a stimulus specific manner indicates that these cells had become a functional part of the visual cortex.
Figure 2: Orientation Specificity of Reprogrammed Cells.
In summary, Tang et al. (2021) were able to show that nonfunctional glial cells surrounding damaged tissue in the primary visual cortex could be converted in into neurons. They then found, using CRACM, that these reprogrammed cells were well integrated into the visual cortex. Finally, using calcium imaging techniques, they found that the reprogrammed cells took on functional properties of primary visual cortex neurons. Together, these findings represent a fascinating and elegant approach to traumatic brain injury therapy.
If you would like to learn more about the tools that Mightex has available to support CRACM techniques, take a look here.
And if you would like to learn more about our tools available to support calcium imaging techniques, take a look here.
Joe Banning, Applications Specialist at Mightex
To read the full publication, please click here.