The invention of optogenetics has enabled scientists to use light to turn cell-type specific neural activity on or off with millisecond-precision to probe neural circuit function (Yizhar et al. 2011). Complementing optogenetics, voltage imaging enables scientists to observe cell-type specific neural circuit activity with cellular-resolution using fluorescent indicators (Knopfel & Song, 2019).
With voltage imaging, scientists can closely replicate electrophysiology measurements, but with the advantage of visualizing large neural populations. Integrating optogenetics with voltage imaging opens the possibility of performing all-optical electrophysiology (Hochbaum et al. 2014).
All-optical electrophysiology would enable scientists to image the activity of large neuron populations and simultaneously perturb cellular activity using optogenetics to both ‘read’ and ‘write’ neural activity. The integration of optogenetics and voltage imaging opens the door to these techniques being performed simultaneously in the same experiment to investigate the causal link between neural circuit activity, function, and behaviour.
To perform all-optical electrophysiology experiments with both voltage imaging and optogenetics, scientists require optimized biology and the appropriate equipment.
All-Optical Biology
A wide-range of optogenetic probes have been developed, differing in function (excitation or inhibition), activation time, and expression properties.
Optogenetic probes are excited by wavelengths of light ranging from blue to red, depending on their properties, and they have only an excitation spectrum to activate their excitation/inhibitory properties (Yizhar et al. 2011).
In contrast, GEVIs behave in the same manner as a fluorophore (e.g. GFP), such that they exhibit excitation and emission spectra. However, the fluorescent signals of GEVIs are dependent on changes in voltage (i.e. increased fluorescence emission) and display dynamic behaviour, unlike a static signal emitted from a fluorophore (Knopfel & Song, 2019).
One problem encountered by combining optogenetics and voltage imaging is overlapping excitation spectra, such that both probes have a similar excitation wavelength. This means that, when trying to image the GEVI, you can potentially activate the optogenetic probe at the same time. Thus, due to potential optical crosstalk, it is not possible to determine if measured changes in the voltage imaging signal are due to natural changes in neural activity or optogenetic-induced changes.
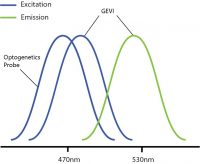
Overlapping excitation spectra of the optogenetics probe and voltage imaging probe.
Optical crosstalk in all-optical electrophysiology experiments can be reduced by selecting imaging indicators and optogenetic probes with non-overlapping excitation spectrums (Emiliani et al. 2015).
An example of this is used by Fan et al. 2020 where they used red-shifted GEVI SomArchon and blue-shifted optogenetic probe CheRiff for all-optical electrophysiology. This decreased the likelihood of crosstalk by reducing or even preventing indirect activation of the optogenetic construct during imaging.
The further optimization of voltage imaging and optogenetics probes (e.g. sensitivity, excitation spectra) will help prevent optical crosstalk in all-optical electrophysiology experiments.
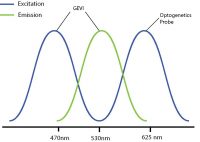
By selecting a wavelength shifted opsin, you can reduce any potential optical crosstalk.
Equipment: Widefield vs. Cellular-Resolution Optogenetics
Once the biology is optimized, you will require the appropriate equipment. For all-optical electrophysiology experiments, there are two options for optogenetics: widefield and cellular-resolution.
Widefield optogenetics uses a standard light source to illuminate the entire field of view. Thus, all neurons will be stimulated indiscriminately, with no ability to select individual neurons.
In comparison, the ability to illuminate select neurons within a population of optogenetic-expressing neurons is called cellular-resolution optogenetics (Shemesh et al. 2017). This method requires more sophisticated spatial specificity to selectively stimulate individual neurons within an optogenetic-expressing population.
The ability to illuminate certain individual neurons is not a limitation of optogenetics per say, but a limitation of the standard optogenetic light sources used in most optogenetic systems. As mentioned above, standard light sources can only illuminate all cells within the field of view, and they have no ability to control where they illuminate to target individual neurons.
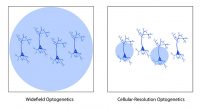
Comparison between widefield and cellular-resolution optogenetic stimulation.
The development of spatially targeted light technologies have enabled researchers to control where the light illuminates the sample, such as an individual cell, making cellular-resolution optogenetics possible (Ronzitti et al. 2017). For example, a digital micromirror device (DMD), such as Mightex’s Polygon Patterned Illuminator, allows researchers to illuminate multiple select individual neurons or regions simultaneously to perform cellular-resolution optogenetics.
Cellular-resolution optogenetics has multiple applications in the field of neuroscience. For example, scientists can study neural circuits and decode neural patterns at the level of individual neurons (Anastasiades et al. 2020; Tran et al. 2019). These types of studies have been carried out both in vitro and in vivo using different technologies (Shemesh et al. 2017; Anastasiades et al. 2020; Tran et al. 2019; Chen et al. 2019). The experimental applications for cellular-resolution optogenetics is endless.
Depending on your all-optical electrophysiology experiment, there are options available for both widefield and cellular-resolution optogenetics integration.