Towards a microcircuit-based understanding of multiplexed spike code in the neocortex
Matthew Tran & Blake Richards
Department of Biological Sciences, University of Toronto
Information about the world is communicated by populations of neurons through action potentials, or “spikes”. Currently there exists two hypotheses about how neurons communicate with each other: (1) through the rate of spikes (rate code) and (2) through the precise timing of spikes (temporal code)1. While evidence suggests that sensory circuits within the neocortex use both rate and temporal synchrony of spikes, i.e. a multiplexed spike code, to transmit information, there lacks a clear mechanistic understanding of how the neocortex could simultaneously use two different coding schemes(1,2,3).
One intriguing possibility could be due to the cellular diversity that exists within the brain. Since Ramón y Cajal’s neuroanatomical drawings in the 19th century, it has been well established that many different cell types exist within the brain. And indeed, over the last decade, a lot of work has been done showing that this cellular diversity can also lead to functional diversity within different neural circuits(4). Thus, we hypothesize that given the diversity of cell types within the neocortex, certain classes of cells, particularly subclasses of interneurons for which many behaviours and mechanisms have been attributed to, may be more or less sensitive to either rate or temporal coding regimes(4).
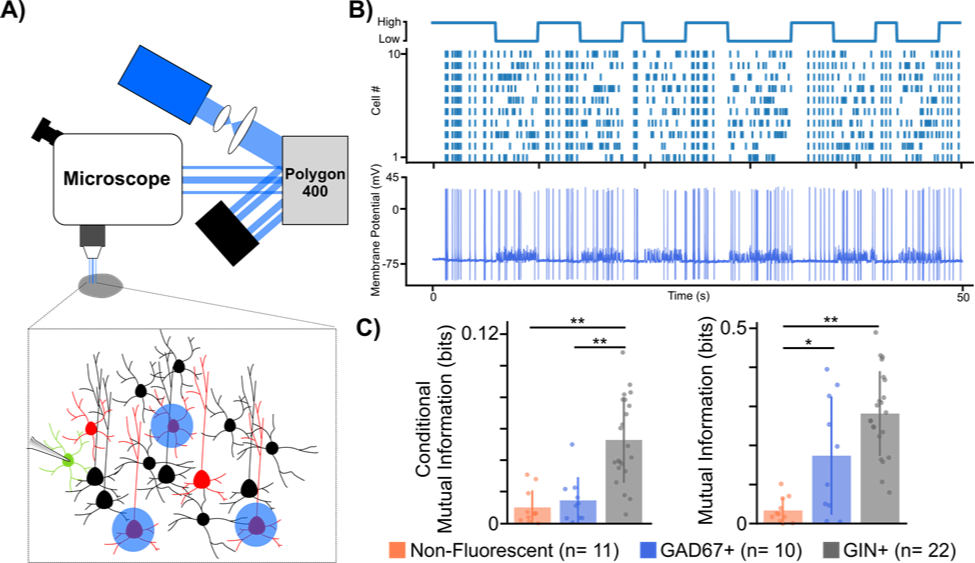
Figure 1. Using a digital micromirror device to study microcircuit multiplexing in the neocortex. A) Experimental set-up using digital micromirror device (Polygon400, Mightex). B) Example trace from GAD67+ cell showing high mutual information to temporal code stimulation. Top: illustration of temporal protocol showing when a high or low temporal code (i.e. synchrony) is induced, Bottom: Example trace from GAD67+ cell to temporal protocol stimulation. C) Mutual information analysis of different cell type spiking responses to both rate (left) and temporal (right) codes. * p < 0.05, ** p < 0.001 Welch’s t-test with Bonferroni correction.
To test our hypothesis, we developed a novel experimental paradigm that biased the spiking patterns of pyramidal cell populations to convey either a rate or temporal code while electrical postsynaptic recordings from either one of two major interneuron types, somatostatin positive (SST+) or parvalbumin positive (PV+), were obtained. To carry out our experiments we used transgenic mouse lines (GAD67 and GIN), which express green fluorescent protein (GFP) in SST+ and PV+ interneurons respectively. We then virally expressed the optogenetic actuator Channelrhodopsin-2 (ChR2) in pyramidal cells found in anatomical layers 2/3 of the somatosensory cortex. After 3 weeks, to allow for sufficient viral expression, acute slices of the somatosensory cortex were prepared to allow for whole cell patch clamp recordings of GFP+ interneurons. Crucial to our experiments was the ability to optically stimulate different pyramidal cells at different times in order to bias them towards a rate or temporal code. To do this, we used a digital micromirror device (Polygon400, Mightex) which allowed us to draw patterns around pyramidal cells we wished to stimulate (Fig 1A,B).
In order to analyze whether these different cell types were more or less sensitive to rate or temporal coding, using the signal we generated and the recorded cellular responses, we analyzed whether the recorded cell’s spiking carried any information about the signal. Mutual information analysis of the post-synaptic spiking responses to both rate and temporal protocols suggested that neocortical interneurons, on the whole, carry more information about rate and temporal coding than non-fluorescent control cells, a majority of which were excitatory pyramidal cells. Furthermore, it seemed that the postsynaptic spiking of SST+ cells carried more information about both rate and temporal codes, whereas spiking responses from PV+ cells only carried information about the temporal code when compared to controls. While our data is still preliminary, the fact that different subclasses of interneurons showed cell type specific differences to each coding regime suggests that interneuron diversity may indeed play an important role in the multiplexing of different spike codes in the brain. This is the first time that anyone has ever demonstrated this and was only possible thanks to our use of the Mightex’s Polygon400.
References:
- Panzeri, Stefano, et al. “Sensory neural codes using multiplexed temporal scales.” Trends in neurosciences 33.3 (2010): 111-120.
- Andrási, Tibor, et al. “Differential excitatory control of 2 parallel basket cell networks in amygdala microcircuits.” PLoS Biology15.5 (2017): e2001421.
- Harvey, Michael A., et al. “Multiplexing stimulus information through rate and temporal codes in primate somatosensory cortex.” PLoS biology 11.5 (2013): e1001558.
- Zuo, Yanfang, et al. “Complementary contributions of spike timing and spike rate to perceptual decisions in rat S1 and S2 cortex.” Current Biology 25.3 (2015): 357-363.
- Tremblay, Robin, Soohyun Lee, and Bernardo Rudy. “GABAergic interneurons in the neocortex: from cellular properties to circuits.” Neuron 91.2 (2016): 260-292.